In the fast-evolving world of automotive technology, understanding the fundamentals of aerodynamics is crucial. Aerodynamic drag is air resistance affecting a car’s movement, impacting speed and efficiency.
Did you know accelerating vehicles facing drag force hamper speed, burn fuel, and diminish fuel efficiency? Approximately 50-60% of total fuel energy is spent to overcome this adverse aerodynamic force.
Reducing aerodynamic drag by 10% improves highway fuel economy by around 5% and city fuel economy by about 2%. Therefore, manufacturers strive to minimize drag for enhanced vehicle performance and efficiency.
This article gets into:
- the basics of automotive aerodynamics and drag coefficient,
- explores the significance of wind tunnel testing and computational fluid dynamics (CFD),
- examines the effects of aerodynamic design on fuel consumption and performance, and
- concludes with practical aerodynamic modifications for improved efficiency.
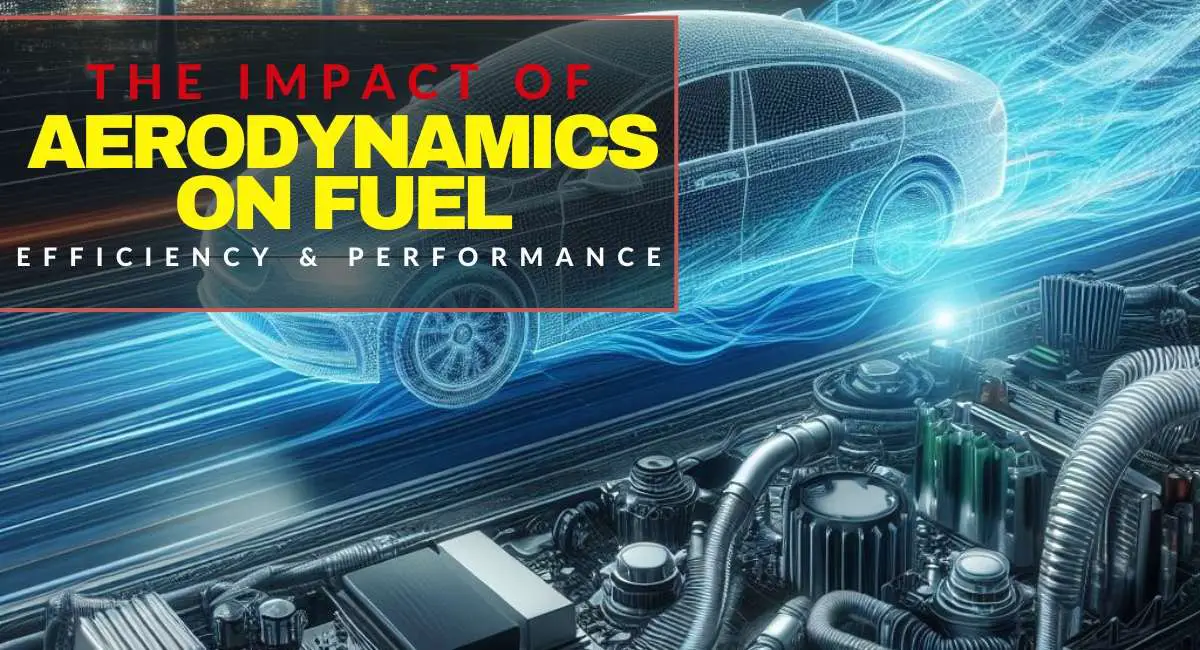
Basics of Automotive Aerodynamics and Drag Coefficient
Automotive aerodynamics is the science of studying how air interacts with vehicles in motion. As your car drives through the air, the air around it can try to slow it down, like wind pushing against it. We call this “drag.” The fundamental principles include reducing air resistance (drag) and improving stability.
Now, the drag coefficient measures how good or bad the car is at avoiding this wind resistance. If the vehicle is shaped well, like a superhero in a streamlined costume, it can cut through the air quickly.
But if it’s not shaped well, like a bumpy costume, it has more trouble moving, and that’s not good for the car’s speed and fuel efficiency. So, making the car more like a superhero helps it go faster and use less energy!
A key metric is the lower the drag coefficient, the more aerodynamically efficient the vehicle is.
Key Principles of Aerodynamics in Vehicles
You need to understand the principles governing aerodynamics. It’s all about reducing air resistance (drag) and improving stability. Modern vehicles have a design that minimizes drag, enhancing fuel efficiency and performance.
So, we have streamlining, a fundamental concept that involves shaping the vehicle to minimize air resistance, allowing for smoother movement through the air. This process is essential for reducing drag and enhancing overall efficiency.
Another crucial principle is downforce, where aerodynamic forces have a creation that improves traction and stability. By manipulating the forces exerted on the vehicle, downforce contributes to better road grip, especially at higher speeds or during dynamic maneuvers.
Conversely, lift management addresses the upward force generated by air passing over the vehicle. Efficient aerodynamic design ensures lift control, preventing instability and promoting a safer and more predictable driving experience.
These principles collectively form the basis for designing vehicles that cut through the air with minimal resistance and maintain optimal stability and control under varying driving conditions.
Comparison of Wind Tunnel Testing and Computational Fluid Dynamics (CFD)
While wind tunnel testing and CFD sound unfamiliar, engineers employ these methodologies to refine aerodynamic designs. It’s time to understand Computational Fluid Dynamics (CFD) and wind tunnel testing.
Wind Tunnel Testing
Wind tunnel testing is a fundamental aspect of automotive aerodynamics. This testing is a whole process that involves several vital steps.
First, there is the creation of a scaled-down model of the vehicle to simulate the aerodynamic behavior on a smaller scale. This model is subjected to controlled wind speeds and directions in a specialized testing environment, typically a wind tunnel. The objective is to replicate real-world aerodynamic conditions and assess how the vehicle performs in different wind scenarios.
You can measure aerodynamic forces during the testing and thoroughly analyze the results. This comprehensive approach allows engineers to gain insights into how the vehicle interacts with airflows, aiding in the refinement of its design for optimal performance and efficiency.
Manufacturers use wind tunnel testing to analyze aerodynamic forces and refine designs for optimal fuel efficiency.
What are the Pros of Wind Tunnel Testing?
The advantages of wind tunnel testing are noteworthy. It provides accurate data on how air interacts with different vehicle components, allowing engineers to decide on design modifications.
There’s more to these advantages, as explained:
- Real-world Simulation: Provides a controlled environment for simulating real-world aerodynamic conditions.
- Precise Measurements: Enables precise measurement of aerodynamic forces acting on scaled-down vehicle models.
- It validates theoretical models and computational simulations, enhancing accuracy.
- Iterative Design Process: Supports an iterative design process, allowing engineers to optimize vehicle designs.
- Study of Complex Interactions: Allows for studying intricate aerodynamic dynamics, enhancing understanding of complex interactions.
- Development of New Technologies: Serves as a platform for developing and testing new aerodynamic technologies.
- Safety Testing: Simulates extreme conditions for assessing vehicle safety and stability under challenging circumstances.
- Industry Standard: Widely accepted as an industry-standard method for evaluating vehicle aerodynamics.
Limitations of Wind Tunnel Testing
Wind tunnel testing has limitations, including:
- In the wind tunnel, precision meets reality, but it comes at a cost – it’s pricier and takes more time.
- Scaling down conditions may introduce inaccuracies. Besides, certain phenomena are challenging to replicate accurately.
- Wind tunnels may not replicate all real-world environmental factors. And so we cannot simulate unsteady flows. For instance, Dynamic flows are challenging to reproduce accurately.
- Model Mounting Influence: Mounting structures can affect results and introduce variables.
- Accessibility: Not all researchers have ready access to wind tunnel facilities.
- Non-linear Scale Effects: Some aerodynamic effects do not scale linearly with size.
- Environmental Impact: Concerns about energy consumption and ecological footprint.
- Today, technology and Computational Fluid Dynamics (CFD) aim to address limitations.
Despite these limitations, wind tunnel testing remains valuable, and technological advancements, along with the integration of computational methods like Computational Fluid Dynamics (CFD), aim to address some of these challenges.
Meanwhile, CFD dances with flexibility and cost-effectiveness, swiftly testing diverse designs in the virtual arena.
Computational Fluid Dynamics (CFD)
CFD is a computerized simulation method that predicts airflow patterns and aerodynamic forces. It enables engineers to analyze complex scenarios without the need for physical models.
Pros of CFD
- Cost-Effective: CFD is generally more cost-effective than traditional wind tunnel testing, reducing the expenses associated with physical model construction and testing.
- Rapid Design Iterations: CFD allows for quick and efficient design iterations, enabling engineers to explore a wide range of design options relatively quickly.
- CFD provides detailed insights into complex fluid dynamics, offering a comprehensive understanding of aerodynamic behaviors, including pressure distribution, turbulence, and flow patterns.
- Simulates Real-world Conditions: CFD simulations can replicate various real-world conditions, such as different wind speeds, angles of attack, and environmental factors, providing a versatile testing environment.
- Accessibility: You can conduct CFD simulations remotely, making them accessible to researchers and engineers worldwide without needing physical testing facilities.
- Environmental Impact: CFD reduces the environmental impact associated with physical testing, as it does not require energy-intensive wind tunnel facilities.
- Flexibility in Boundary Conditions: CFD allows for flexibility in setting boundary conditions, enabling simulations of various scenarios and driving conditions.
Limitations of Computational Fluid Dynamics (CFD)
- Accuracy Challenges: Achieving high accuracy in CFD simulations can be tricky, and the outcomes may vary based on the quality of the mesh, turbulence modeling, and numerical methods used.
- Computational Resources: CFD simulations demand significant computational resources, and complex simulations may require high-performance computing (HPC) facilities, leading to increased costs.
- Validation Required: CFD results need validation against experimental data to ensure accuracy, and discrepancies may arise between simulated and real-world conditions.
Complexity in Model Setup: Setting up a CFD model requires expertise, and the simulation’s complexity increases with the design’s intricacy and the desired level of detail. - Limited Physical Interaction: Unlike wind tunnel testing, CFD lacks physical interaction with a tangible model, potentially missing specific dynamic effects or unexpected phenomena.
- Initial Learning Curve: Using CFD software requires a learning curve, and obtaining accurate and meaningful results necessitates experience and expertise.
- Boundary Condition Challenges: Accurately defining boundary conditions, such as inlet and outlet conditions, can be challenging and may impact the reliability of the simulation.
- Data Post-processing: Extracting meaningful information from CFD results involves sophisticated post-processing, adding complexity to result interpretation.
CFD has become increasingly prevalent in the automotive industry due to its cost-effectiveness and ability to simulate various conditions accurately.
Comparing wind tunnel testing and CFD reveals a synergy between the two. While wind tunnel testing provides empirical data, CFD offers a more flexible and cost-efficient approach to exploring various design variations.
Effects of Aerodynamic Design on Fuel Consumption and Performance
Fuel Efficiency
Aerodynamics plays a pivotal role in fuel efficiency. The reduction of drag directly contributes to lower fuel consumption. Studies indicate that for every 10% decrease in drag coefficient, there can be an approximate 5-7% improvement in fuel efficiency, highlighting aerodynamic design’s economic and environmental benefits.
Performance Impact
Beyond fuel efficiency, aerodynamics significantly influences vehicle performance. A well-designed aerodynamic profile enhances acceleration, top speed, and stability. Manufacturers invest in aerodynamic advancements to meet fuel economy standards and deliver a superior driving experience.
Real-world examples showcase the impact of aerodynamic design on performance. High-performance vehicles often feature sleek, aerodynamic shapes to achieve top speeds and optimal handling.
Practical Aerodynamic Modifications for Improved Efficiency
Practical aerodynamic modifications offer enthusiasts and manufacturers the opportunity to enhance vehicle efficiency. As the vehicle becomes efficient, then more fuel is conserved. Body kits, spoilers, and underbody panels are popular modifications.
Body kits have a design that alters the vehicle’s appearance while improving aerodynamics. Spoilers help manage airflow, reducing lift and enhancing stability. Underbody panels streamline the airflow beneath the vehicle, minimizing drag.
Additionally, year diffusers have a design that accelerates the airflow under the vehicle, reducing air pressure and further minimizing drag. It is prevalent in high-performance and sports cars. Plus, some advanced suspension systems can automatically adjust ride height and stiffness based on driving conditions, contributing to improved aerodynamics.
Venting in the wheel arches helps manage turbulent air around the tires, reducing drag and enhancing aerodynamic performance. We have the rear spoilers and wings design, which manages airflow around the vehicle, providing downforce to improve traction and stability, especially at higher speeds.
Research indicates that well-executed modifications can lead to notable improvements in aerodynamic efficiency. However, balancing aesthetics and functionality is crucial, ensuring that modifications serve both purposes effectively.
Case Studies
Examining real-world examples reinforces the impact of aerodynamics on fuel efficiency and performance. Vehicles like the Tesla Model S and the Toyota Prius exemplify how aerodynamic design contributes to their remarkable fuel efficiency.
Data from these case studies demonstrates tangible results. For instance, the Toyota Prius
has a compact front area and a small upper grille that diverts air around the body instead of through it.
A subtle chin spoiler beneath the bumper and flat front bumper corners help manage airflow around and under the car, reducing turbulence in the wheel arches.
Future Trends and Innovations
As technology advances, future trends in automotive aerodynamics are worth exploring. Emerging technologies such as active aerodynamics, which adjusts vehicle components based on driving conditions, promise even more significant gains. Some current and future trends that’ll stick include Integrated rear spoilers, active grille shutters, and contoured body lines crafted to fulfill both aerodynamic functions and aesthetic appeal.
Innovations in materials, such as lightweight yet durable composites, contribute to improved aerodynamic performance. As electric vehicles become more prevalent, designers are reimagining vehicle shapes to optimize aerodynamics and extend range.
Conclusion
The impact of aerodynamics on fuel efficiency and performance is undeniable. The basics of automotive aerodynamics pave the way for a future where vehicles are more fuel-efficient and offer an exhilarating driving experience.
Practical aerodynamic modifications allow enthusiasts to customize their vehicles while reaping the benefits of enhanced efficiency. As we look ahead, future trends and innovations in automotive aerodynamics promise an exciting era of even more significant advancements. In the pursuit of sustainable and high-performing vehicles, the role of aerodynamics remains at the forefront of automotive engineering.